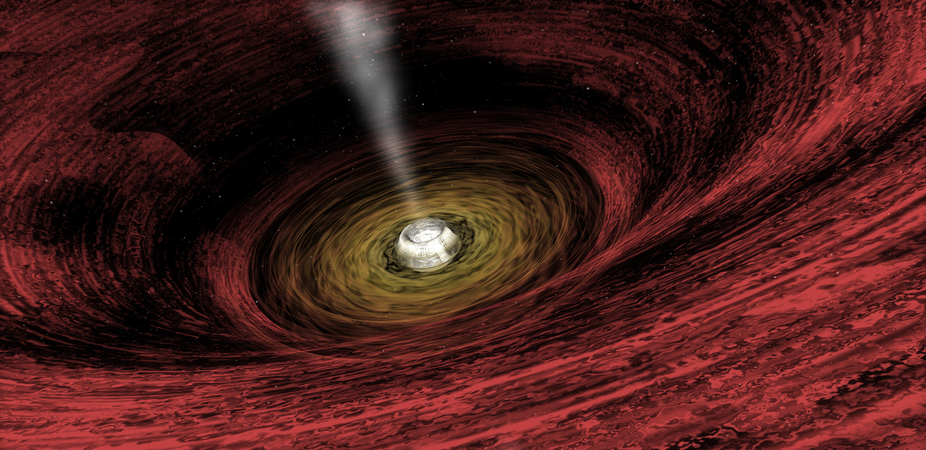
This composite image combines the deepest X-ray image ever taken with optical and infrared data from Hubble. Astronomers obtained what is known as the Chandra Deep Field South by pointing the Chandra telescope at the same patch of sky for over six weeks of time. The Chandra sources of this small section of the CDFS are shown in blue. Two "stacked" images, which represent a technique used to find the most distant galaxies in X-ray light, are on the right. The results from this dataset include that black holes are found to be actively growing between 800 million and 950 million years after the Big Bang.
New research shows supermassive black holes are bigger than the sum of their parts. Image: NASA/CXC/A.Hobart
By Vikram Ravi, University of Melbourne and Ryan Shannon, Astronomy and Space Science, CSIRO
Galaxies may look pretty and delicate, with their swirls of stars of many colours – but don’t be fooled. At the heart of every galaxy lies a supermassive black hole, including in our own Milky Way.
Black holes in some nearby galaxies contain ten billion times the mass of our sun in a volume a few times the size of our solar system. That’s a lot of mass in a very small space – not even light travels fast enough to escape a black hole’s gravity.
So how did they get that big? In the journal Science, we tested a commonly-held view that black holes become supermassive by merging with other black holes – and found the answer is not quite that simple.
Searching for gravitational waves
The answer may lie in a related question: when two galaxies collide to form a new galaxy, what happens to their black holes?
When galaxies collide, they form a new, bigger galaxy. The colliding galaxies’ black holes sink to the centre of this new galaxy and orbit each other, eventually combining to form a new, bigger black hole.
Black holes, as the name suggests, are very hard to observe. But orbiting black holes are the strongest emitters in the universe of an exotic form of energy called gravitational waves.
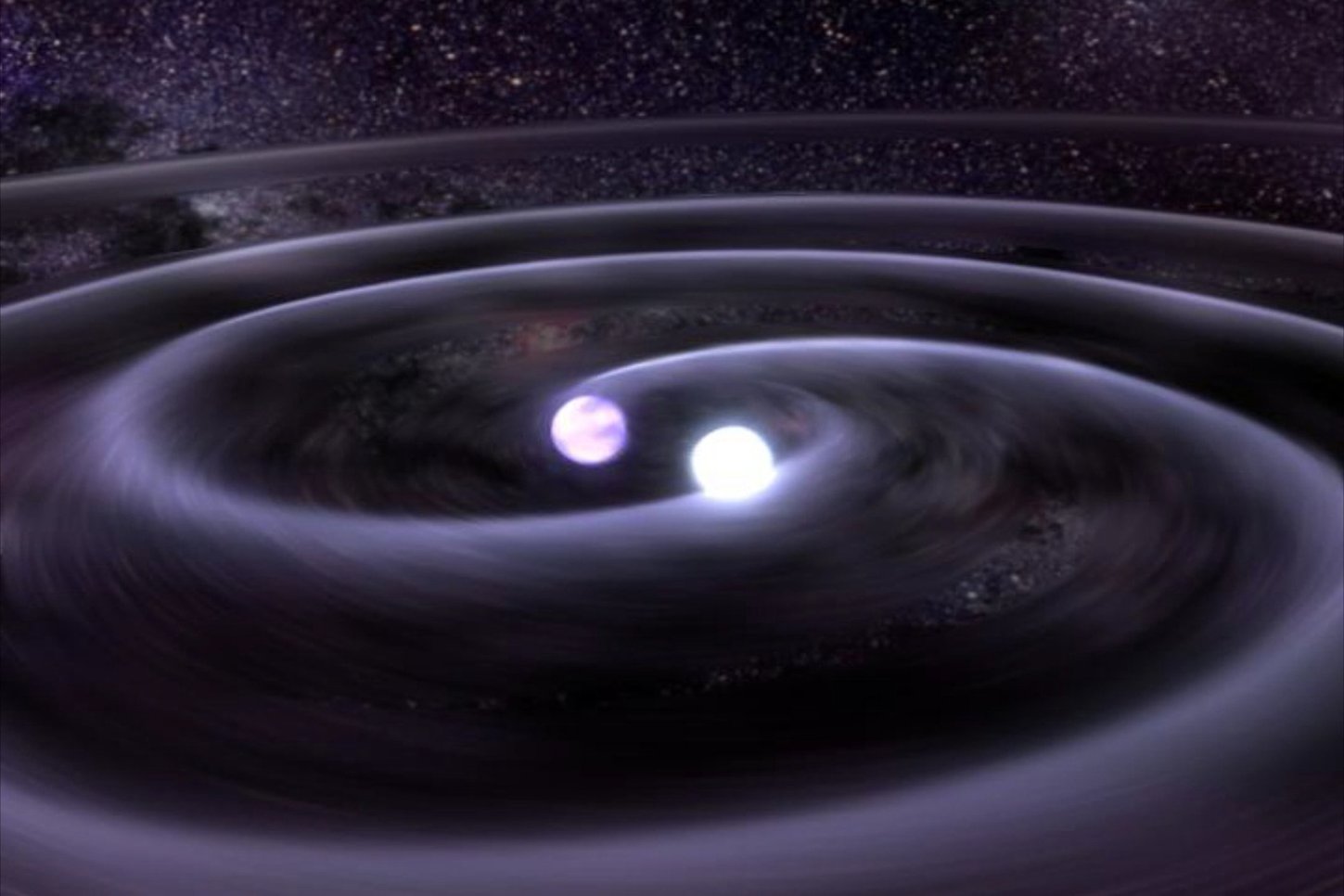
Orbiting black holes generate gravitational waves. Image: NASA
Gravitational waves are a prediction of Einstein’s General Theory of Relativity and are produced by very massive, compact objects changing speed or direction. This, in turn, causes the measured distances between objects to change.
For example, a gravitational wave passing through your computer screen will cause it to first stretch in one direction, then in a perpendicular direction, over and over again.
Fortunately for your laptop, but unfortunately for astronomers, gravitational waves are very weak. Gravitational waves from a pair of black holes in a nearby galaxy causes your screen size to change by one atomic nucleus over ten years.
But fear not – a way to detect these waves exists by using other extreme astronomical objects: pulsars, which are leftovers of massive stellar explosions called supernovae.
While they’re not quite as extreme as black holes, pulsars are massive and compact enough to crush atoms into a sea of nuclei and electrons. They compress up to twice the mass of our sun into a volume the size of a large city.
So how do pulsars help? First, they rotate very quickly – some of them up to 700 times per second – and very predictably. They emit intense lighthouse-like beams of radio waves, which, when they sweep by the Earth, appear as regular “ticks” – see the video below.
So here’s the punchline: gravitational waves from pairs of black holes throughout the universe will disrupt the otherwise extremely regular ticks from pulsars in a way we can measure.
Our pulsar measurements
We found that the theory that black holes grew mainly by absorbing other black holes is not consistent with our data.
If the theory was right, gravitational waves would exist at a level that would cause the ticks to appear less regularly than our measurements. This means that black holes must have grown by other means, such as by consuming vast swathes of gas churned up during galaxy mergers.
We used measurements of pulsar ticks from the CSIRO Parkes Radio Telescope (the Dish) collected by the Parkes Pulsar Timing Array project led by the CSIRO and Swinburne University of Technology.
The measurements span over ten years, and are some of the most precise in existence.
These data are being collected to eventually directly observe gravitational waves. In our work, however, we compared the data with gravitational wave predictions from various theories for how black holes grew.
Our work gives us great encouragement for the prospects for using pulsars to detect gravitational waves from black holes.
We are confident that gravitational waves are out there – galaxies, after all, do collide – and we have shown that we can measure pulsar ticks with sufficient accuracy to be able to detect gravitational waves in the near future.
In the meantime, we can even use the absence of gravitational waves to study elusive super-massive black holes.
This article was originally published at The Conversation. Read the original article.
7th November 2013 at 6:37 pm
Nice article! A fascinating topic and good to hear of the amazing research being carried out in this country.
30th October 2013 at 11:14 am
Reblogged this on Telescopes in Schools and commented:
Research done by TiS volunteer, Vikram Ravi and colleagues. Well done Vikram on some amazing research.